How does air pollution from marine shipping accelerate climate change in the Arctic and what can be done about it?
Getting your Trinity Audio player ready...
|
An increasing number of ships in Arctic waters, lured by the hope of faster and more direct routes between the Pacific and Atlantic oceans, present a threat to the region and the world, due to a poorly understood phenomenon.
Nearly invisible sooty particles of engine exhaust – black carbon – drift out of the sky onto polar ice and snow. The exhaust is produced from commercial ships sailing locally as well as ships and other sources1 thousands of kilometres away. When this combustion by-product collects on polar ice and snow, they darken, warm, and melt. If unchecked, this phenomenon poses a severe risk not only to the pristine Arctic environment and all that depend on it for life, but also to the entire planet from accelerated climate change and increasing global temperatures.
Black carbon emissions from ships have grown ten times faster in the Arctic compared to the rest of the world, with an 85% increase in black carbon emitted by ships in the Arctic between 2015 and 2019, compared to an 8% increase globally2. However, black carbon has yet to be addressed by international or national climate change regulations, and scientists are still working to understand its potential to cause harm in the Arctic and the global climate system.
Navigating Canada’s relatively uncharted northern waters and finding ways to limit or eliminate sources of black carbon will take work. This important work begins with understanding black carbon’s properties, how it behaves in Arctic conditions and how the marine shipping industry can reduce its emissions of an air pollutant that threatens both people and the environment in the Arctic.
This article aims to unravel the complexities of black carbon as a climate pollutant and inform policies intended to protect and support Canada’s Arctic communities, economies, and environment.
What is black carbon and why is it a problem?
Black carbon is a carbon-based aerosol produced by the incomplete combustion of fossil fuels, biofuels, and biomass.3 A component of fine particulate matter (PM2.5),4 black carbon absorbs light and heat. It is categorized as a short-lived climate pollutant (SLCP), existing for a shorter time than carbon dioxide (CO2) once released into the atmosphere – typically days to weeks. After CO2, black carbon is the second-leading contributor to global warming, but its effect is a complicated twist on the traditional greenhouse theory many of us are familiar with.
Imagine wearing a black shirt or a white one during the summer; the dark cloth absorbs the sun’s energy, making the wearer warmer, while the white reflects. Similarly, ice and snow reflect the sun’s heat back to the atmosphere while darker surfaces absorb the sun’s rays. This is known as the albedo effect. Black carbon particles do just that; they absorb the sun’s radiation and heat up what is around them, melting ice and exposing darker seawater or land underneath. This can lead to a vicious cycle of warming and melting that gets worse over time.
But the full picture is more complex. In certain conditions black carbon can have a cooling impact. Black carbon particles emitted at high levels of the atmosphere, such as from planes (known as nvPM in the aviation industry), interfere with cloud formation, and depending on weather and cloud position, can stabilize a layer of air atop the clouds. These clouds will block or scatter sunlight and therefore protect the underlying surface from heating up. The particles themselves can also reflect the sun’s radiation. However, scientists have found that the warming effects of black carbon outweigh the cooling effects, creating a problem.
What is the impact of black carbon in the Arctic?
The effect of black carbon is complex, as black carbon particles emitted from outside of the Arctic and transported into the region via weather systems may interact with cloud formation, initially having a cooling impact. Then black carbon absorbs solar energy and warms the atmosphere while floating in the air. However, some airborne black carbon particles will eventually land on ice or snow.
Conditions in the Canadian Arctic, with vast tundra, snow-covered landscapes, and expansive sea ice, are particularly susceptible to the effects of black carbon. When black carbon particles settle on ice or snow, they change the surface’s colour and its ability to reflect light (albedo). This reduced albedo is one reason why the Arctic is warming more than two times faster than the rest of the globe.6
Now covered in a layer of black carbon, the ice or snow surface absorbs more of the sun’s radiant energy, accelerating melt. This direct warming impact adds to the atmospheric warming created by floating black carbon particles.7 As the warming ice melts, it exposes dark water that absorbs more of the sun’s heat energy than ice or snow, further accelerating the warming process.
In the Arctic, the warming effects of black carbon outweigh the cooling effects from upper atmosphere particles as the majority of black carbon particles are emitted from sea-level sources within the region, such as ships that are operating close to glaciers, snow-covered coasts, or sea ice edges.8
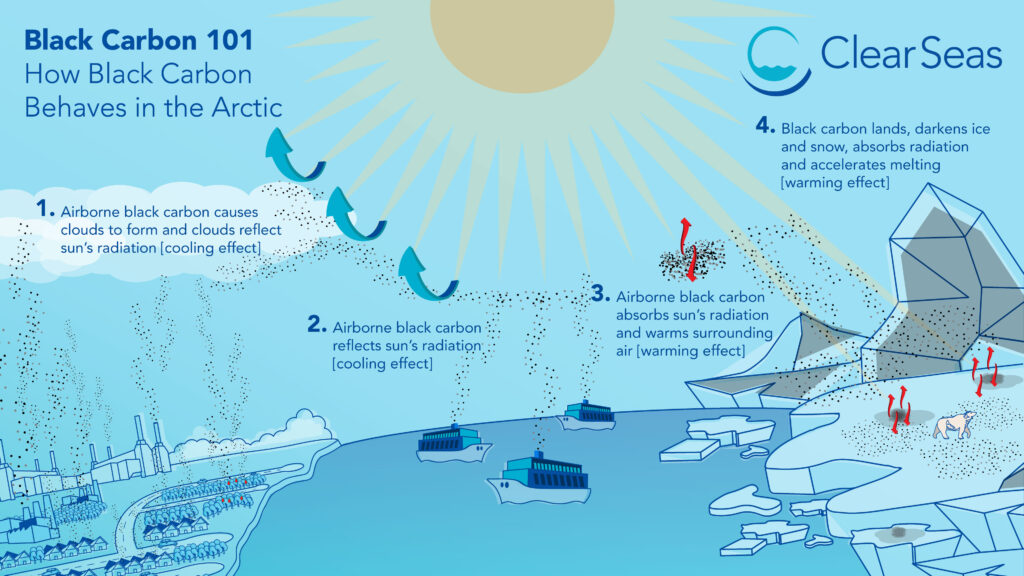
How does black carbon impact human health?
Climate warming is not the only black carbon impact of concern in the Arctic. Despite its short atmospheric lifespan,9 black carbon has adverse health effects on humans. Black carbon particles contribute to heart, circulatory and respiratory diseases. The United Nations Environment Program’s 2012 study on the health impacts of black carbon revealed it to be a universal carrier of chemicals of varying toxicities, affecting the lungs, the body’s major defence cells and possibly systemic blood circulation.10 For Indigenous and coastal communities that rely on subsistence hunting and locally sourced foods, the health risks of black carbon can be even higher; as ice-reliant polar bears and seals struggle to survive in a changing climate, so too will people for whom these animals are an essential food source.11 Further research is needed to understand the short- and long-term effects of black carbon exposure on human health.
Where does black carbon come from?
Black carbon comes from natural and human sources. In the Arctic, some black carbon is produced naturally through wildfires. Arctic wildfires come in many forms, but the most problematic are “zombie fires”.12 Zombie fires can thaw permafrost while smouldering under snow or ice13 and emit CO2 and black carbon particles once the snow and ice melt and the fire erupts on the surface.
Various human activities produce black carbon, from household cooking fires to commercial fuel combustion and resource extraction processes such as flaring waste gas.14 Commercial ship exhaust accounts for a growing share of black carbon particles in the Arctic.15
How does the maritime industry contribute to black carbon emissions in the Arctic?
Ships are the only source of black carbon emitted at sea-level through or near sea ice. Ships remain the most affordable and efficient way to transport goods despite their reliance on fuels that emit black carbon particles.
Marine shipping is vital to the Arctic region’s survival, supplying remote communities and industrial sites with goods and services during the summer months. However, the growth of global trade; growth in Arctic oil, gas, and mining operations; and the increasing viability of trans-Arctic shipping require black carbon abatement measures to halt sea-ice loss and protect coastal communities and the environment from further harm.
Sea ice loss from a warming climate does not immediately mean smoother sailing. Changing weather and climate conditions in the Arctic have created more variable and volatile sea ice resulting in increased risks to ships and associated delays in transit. If a ship is not sufficiently strengthened (categorized as ice-class), it must be accompanied by an icebreaker for safety. Ice thickness, concentration and drift speed and direction are all factors that impact travel time and fuel consumption; while slower moving ships burn less fuel in ice-free waters, ice conditions increase resistance, meaning more fuel must be burned to maintain speed.16 Another consideration is that a ship’s journey may be significantly prolonged as it waits out changing Arctic weather conditions; the more time at sea, the more fuel burned – and the more black carbon and other air emissions produced.
Ship operating conditions in the Canadian Arctic remain variable due to many factors, especially the navigational challenges posed by a lack of infrastructure and changing weather and ice conditions. Future fuel consumption and associated emissions are difficult to predict, and therefore challenging to mitigate. At present, shipping traffic contributes about five percent of black carbon emissions in the Arctic17 due to Arctic waterways’ limited accessibility. However, as shipping through the Arctic becomes more widespread, experts predict that black carbon emissions throughout the region could triple by 2030 without regulations to limit emissions.18
How are black carbon emissions from ships in the Arctic being addressed?
Given black carbon’s complexities and potential impacts on the Arctic, many local and international groups are working to address black carbon emissions. The Arctic Council’s Fairbanks Declaration in 2017 is the first pan-Arctic goal to reduce black carbon emissions from a range of sources by 25% to 33% below 2013 levels by 2025. This collective, aspirational goal is supported by all Arctic States and in 2021, the Expert Group on Black Carbon and Methane found that Arctic States have reduced their collective black carbon emissions by 20% in 2018 and are on track for 2025. However, fuel consumption for marine shipping in the Arctic grew 82% between 2016 and 2019, threatening future collective black carbon reductions.19
The Arctic Council and the International Maritime Organization (IMO) have groups working collaboratively to understand and respond to black carbon emission impacts, including the IMO’s Sub-Committee on Pollution Prevention and Response (PPR).
The regulation of black carbon emissions from ships is further complicated by a lack of sampling and measurement standards. Canada has established an international Technical Working Group to develop a standardized sampling and measurement protocol for black carbon emissions from marine engines and studies are underway to enable accurate and comparable measurements.
The PPR has made some progress on understanding how better fuel options could lead to reductions in black carbon emissions.20 Heavy fuel oil (HFO) is the cheapest available petroleum-based fuel used by commercial ships. HFO is a persistent oil that is extremely difficult to clean in the event of a spill, with the potential to get trapped in and under Arctic sea ice.21 Some HFO is also high in aromatic content that encourages the formation of black carbon when it is burnt. Moves to reduce the sulphur content of HFO, for example the global sulphur cap on marine fuels implemented in 2020, could have reduced black carbon emissions if had it resulted in the use of lower aromatic fuels; however, evidence to date suggests that some low-sulphur fuels can have similar aromatic content as HFO.
Particulate filters that remove 90% or more of black carbon from the exhaust could be developed for use in the marine environment.22, 23 Filters are already used for heavy-duty trucks.
Plans are also underway to ban the use and carriage for use of HFO from Arctic waters because of the risk of spills, although the carriage of HFO cargoes will still be allowed as well as ships with certain waivers and exemptions to use HFO. 24
Does the proposed Heavy Fuel Oil Ban reduce black carbon emissions?
The IMO has proposed a ban on the use and carriage for use of HFO in the Arctic.25 This measure seeks to reduce the impact of an oil spill by stipulating that ships must use alternative (less dense) fuels that will evaporate or dissipate in the event of a spill. These alternative fuels must have a density limit below most HFOs (that is, less than 900 kg/m3 at 15°C).
A perceived benefit of the HFO ban is the potential reduction of black carbon and other air pollutants. Fuels such as liquefied natural gas (LNG) and marine diesel (distillate fuel) are current options. The IMO’s Marine Environment Protection Committee moved to ban the use and carriage of HFO in the Arctic Ocean in late 2020, with plans to formally adopt the ban in 2021.26
Despite its potential to improve air quality and reduce oil spill risks, the HFO ban has been met with criticism, and many question what its impact will be over the next decade. As currently worded, the ban will not come into effect until 2024 at the earliest. Through various allowances in the language of the ban, vessels will still be able to use and carry HFO throughout the circumpolar Arctic until mid-2029, leaving the region vulnerable to oil spills and exposed to continued black carbon pollution during this period. In fact, the exemptions and waivers included in the HFO ban regulation are expected to reduce the use of HFO in the Arctic by only 16%, reducing black carbon by only 5%, whereas a full ban on HFO could reduce black carbon emissions by 30%.27
Even once implemented, the HFO ban may not adequately protect the Arctic from climate pollutants like black carbon. There is no guarantee that fuels meeting the criteria set out by the IMO’s regulations banning HFO will be low in aromatics, nor that they will reduce particulate matter emissions like black carbon. Fuels with higher aromatics have poorer combustion, resulting in more black carbon particles emitted.
If sulphur in fuels produces particulate matter, why not regulate sulphur to curb black carbon?
Many different fuels produce black carbon, including potential alternatives to HFO, such as very low sulphur fuel oil. Although sulphur in fuels contributes to the creation of particulate matter, reducing sulphur content may not reduce black carbon emissions. Low sulphur fuel blends, while reducing sulphur oxides emissions, may actually produce more black carbon.28 Some new fuel blends designed to meet IMO’s 2020 0.50% sulphur rule have higher aromatic contents, which result in higher black carbon emissions when burned than low-aromatic distillate fuels such as marine gas oil. A Finland-Germany joint study confirmed a correlation between higher aromatics and higher black carbon and has flagged aromaticity as an issue of concern at the IMO, with a call for more research to better inform policy decisions.29
The IMO 2020 sulphur cap seeks to reduce sulphur emissions from ships by limiting the sulphur content in fuels to 0.5% by mass. More stringent Sulphur Emissions Control Areas (SECA) are in place around the coasts of North America and Europe, limiting sulphur to 0.1%, but the Arctic was not included in the North American SECA.
Further, the IMO 2020 regulations to limit sulphur do not eliminate the risk of a persistent oil spill. The Wakashio spill in Mauritius in 2020 is one example of the harm caused by very low sulphur fuel oil. A spill of a similar nature would be further complicated under Arctic conditions due to the potential for oil to become trapped under ice. Many low sulphur fuels still contain a certain amount of persistent oil, and ship operators are permitted to continue using HFO and other high-sulphur fuels if they install an exhaust gas cleaning system (commonly known as a “scrubber”).
Scrubbers may reduce black carbon as well as sulphur oxides for ships that continue to use HFO. Scrubbers clean sulphur oxides from ship exhaust before the exhaust is released to the atmosphere, and preliminary research suggests that scrubbers might help efforts to reduce black carbon from ships using high sulphur HFO, but ships using low-sulphur fuels are expected to emit less black carbon than ships using scrubbers.30 Scrubber use also raises water pollution concerns since the sulphur and other contaminants removed from the exhaust are usually discharged overboard in the form of acidic washwater.31
Should particulate emissions from ships be directly regulated?
In all other forms of transport – road, rail, and inland waterways – particulate matter emissions are limited through air quality regulations. While important mechanisms for the protection of the global environment, the IMO’s regulations to limit sulphur and ban HFO in the Arctic only target certain aspects of some marine fuels and may not effectively reduce black carbon emissions in the Arctic.
Environmental groups worldwide are advocating for regulations to directly address black carbon emissions. The current regulations for polar regions, the IMO International Code for Ships Operating in Polar Waters or Polar Code, make no mention of ship-source air pollution or greenhouse gases.
Untangling the contradictions to better inform policy
As a short-lived climate pollutant, black carbon’s limited atmospheric lifetime means that abatement measures, if correctly executed, will reduce black carbon emissions almost immediately, helping to prevent additional near-term Arctic and global warming. When discussing climate change in the circumpolar Arctic, there is no shortage of contradictions.
Emissions from ships contribute to climate change. The loss of sea ice from global warming creates new possibilities for Arctic shipping. Untangling such contradictions is challenging but doing so allows science to inform policy.
Thoughtful, evidence-based policy will allow the Canadian Arctic’s communities and environment to be better protected from harmful pollutants like black carbon while still benefiting from the economic opportunities increased vessel traffic may offer.
References
1 For example, industrial, commercial, and residential fuel uses as well as forest fires.
2 Comer, B. (2021). Switching fuel: how to cut black carbon emissions from Arctic shipping.
3 Climate and Clean Air Coalition. (2021). What are short lived climate pollutants?
4 Fine inhalable particles, with diameters that are generally 2.5 micrometers and smaller.
5 Bachmann, J. (2009). Pew Centre on Global Climate Change: Black carbon: A science/policy primer.
6 Arctic Monitoring and Assessment Program. (2021). EU-funded action on black carbon in the Arctic.
7 Arctic Monitoring and Assessment Program. (2011). The impact of black carbon on Arctic climate.
8 Arctic Monitoring and Assessment Program. (2021). EU-funded action on black carbon in the Arctic.
9 In comparison to black carbon’s short atmospheric lifespan of a few days to weeks, CO2 has an atmospheric lifespan of 5 to 200 years. Source: IPCC Working Group 1: The Scientific Basis.
10 United Nations Environment Program. (2012). The health effects of black carbon.
11 Arctic Monitoring and Assessment Program. (2004). Arctic Climate Impact Assessment.
12 World Economic Forum. (2020). Zombie fires and other strange events are happening in the Arctic.
13 A thick subsurface layer of soil that remains frozen throughout the year, occurring chiefly in polar regions (Oxford English Dictionary).
14 Environment Canada. (2021). Black carbon inventory emissions 2021.
15 International Council on Clean Transportation. (2021). Black carbon from ships.
16 Schroder et al. (2017). Environmental impact of exhaust emissions by Arctic shipping.
17 Arctic Council Expert Group on Black Carbon and Methane. (2017). Summary of progress and recommendations 2017.
18 Arctic Monitoring and Assessment Program. (2015). Arctic climate issues 2015 short-lived climate pollutants summary for policy-makers.
19 Expert Group on Black Carbon and Methane (2021). 3rd Summary of Progress and Recommendations, p. 24-25.
20 Sub-Committee on Pollution Prevention and Response (2020). Prohibiting the use and carriage for use as fuel of heavy fuel oil by ships in the Arctic waters-draft MARPOL amendments agreed.
21 Protection of Arctic Marine Environment (PAME). (2019). Heavy fuel oil (HFO) use by ships in the Arctic 2019.
22 Merkisz J., Pielecha J. (2015). Methods of Decreasing Emissions of Particulate Matter in Exhaust Gas.
23 International Council on Clean Transportation. (2019). Sixth ICCT Workshop on Marine Black Carbon Emissions: Black Carbon Control Policies.
24 Sub-Committee on Pollution Prevention and Response (2020). Prohibiting the use and carriage for use as fuel of heavy fuel oil by ships in the Arctic waters-draft MARPOL amendments agreed.
25 International Council on Clean Transportation. (2020). The International Maritime Organization’s proposed Arctic heavy fuel oil ban: likely impacts and opportunities for improvement.
26 High North News. (2020). IMO and Arctic states face criticism over weak HFO ban.
27 International Council on Clean Transportation. (2020). The International Maritime Organization’s proposed Arctic heavy fuel oil ban: likely impacts and opportunities for improvement.
28 International Council on Clean Transportation. (2019). Sixth ICCT Workshop on Marine Black Carbon Emissions: Black Carbon Control Policies.
29 IMO Sub Committee on Pollution and Prevention Response. (2019). Reduction of the impact on the Arctic of black carbon emissions from international shipping.
30 International Council on Clean Transportation. (2021). Global scrubber washwater discharges under IMO’s 2020 fuel sulfur limit.
31 International Council on Clean Transportation. (2020). Air emissions and water pollution discharges from ships with scrubbers.